During the middle ages, the citizens of Italy used to succumb to a mysterious disease that struck in summers, peaking in August and September. The disease seemed to afflict people at random, caused terrible fevers, and was often fatal. It didn’t discriminate between the rich, the poor, the migrant workers, or even the clergy. When the cardinals gathered together to elect a new pope in late summer of 1623, the mysterious plague killed a number of them, along with their attendants.
A review published by the University of Northern Iowa in 1824, stated that “from the Vatican to the Lateran, and from the Villa Borghese to the gate of St Paul, there is no longer any considerable space from which those, who are able, do not escape during the hot months, and only very small portions, where, from some unexplained cause, this mysterious pestilence has not yet intruded.” Even the Romans, known for their excellent record keeping, couldn’t determine the exact number of lives the disease claimed, “because the persons employed here in cultivating the soil do not live on it permanently.”
While the pestilence spread over large areas of Rome and beyond, it seemed to have no obvious source. Yet, one area that caused suspicions was the Pontine Marshes southeast of Rome, located between the Tyrrhenian Sea and the Apennine foothills. Earlier on, it called the Pontine Fields, but after the trees had been cut down, the area became swampy and full of stagnant water. The marshes filled the air with unpleasant smells and the south western winds, which were supposedly common in late summers, brought the smells to the capital. At the time, many medics subscribed to the miasma theory of disease, according to which bad air, called mal’aria in Italian, could cause such ailments. Contrary to what we know now, malaria was believed to be “a dense exhalation chiefly from the Pontine marshes.” So to avoid the noxious fumes, some residents built their houses with few or no windows facing southwest.
During the times of ancient Rome, the Pontine Marshes censor Appius Claudius built the Appian Way through the marshes, draining parts of them, but later the road fell into disrepair. In the 18th century, Pius VI, who believed that the marshes were at fault, attempted to “reduce them, at once, to a state fit for cultivation.” The endeavor consumed a lot of lives, yielded “a magnificent road twenty four miles long, accompanied, like the Appian way, by an ample canal,” but the rest of the marshes were still there—about 100 square miles, according to the historical documents of the time. “And of the remainder a large proportion is still under water. The whole is as much subject as ever to exhalations.”
Not everyone blamed the marshes for malaria—primarily because Italy’s other regions, far from the marshes’ toxic exhalations, got the disease too. Some skeptics pointed out that the marshes were 40 miles away from Rome—could the poisonous air really travel that far? Another theory hypothesized that the noxious gas originated from the volcanic materials of the soil, which after decaying for thousands of years, would finally reach a toxic point “under the influences of the summer’s heat and the action of the sea air.” But whether stemming from the marshes or the soil, it is the air that was thought to be at fault.
Other countries believed the miasma theory too. Philadelphia’s epidemic of yellow fever in 1793 was blamed on the rotting cargo from the West Indies, which led to quarantining bags of coffee beans. The nineteenth century Europeans deemed parts of the Caribbean dangerous because “when a meridian sun unites with a marshy rotten soil, in which the heavy rains stagnate, then it is impossible for a country to be tolerably healthy.”
Yet, despite not getting it exactly right, the medics weren’t that far from the truth. The stagnant water creates breeding grounds for mosquitoes, including the malaria-carrying species. The scientists at the time just didn’t have the tools to identify the actual cause and the correct transmission route—via an infected insect’s bite. It took several researchers to crack that mystery.
Joining The Efforts
One of the first scientists to challenge the miasma theory was French military surgeon Alphonse Laveran. He wrote that not all marshy areas were malaria-ridden and not all malaria hotspots were swampy. There had to be something else at play. In his 1875 “Treatise on Military Diseases and Epidemics,” he suggested that “swamp fevers are due to a germ.”
He proved that theory five years later. In November 1880, at a military hospital in Constantine, Algeria, he saw a malaria parasite, Plasmodium, moving within a red blood cell obtained from a malarial patient. Later that month, A few weeks later, his findings were presented at the Academy of Medicine in Paris. The statement was brief—it read “Dr. Laveran submits a note about a new parasite found in the blood of several patients suffering from swamp fever.” But that short sentence helped revolutionize the entire theory of disease. The miasma concept faded, yielding to the modern-day germ theory.
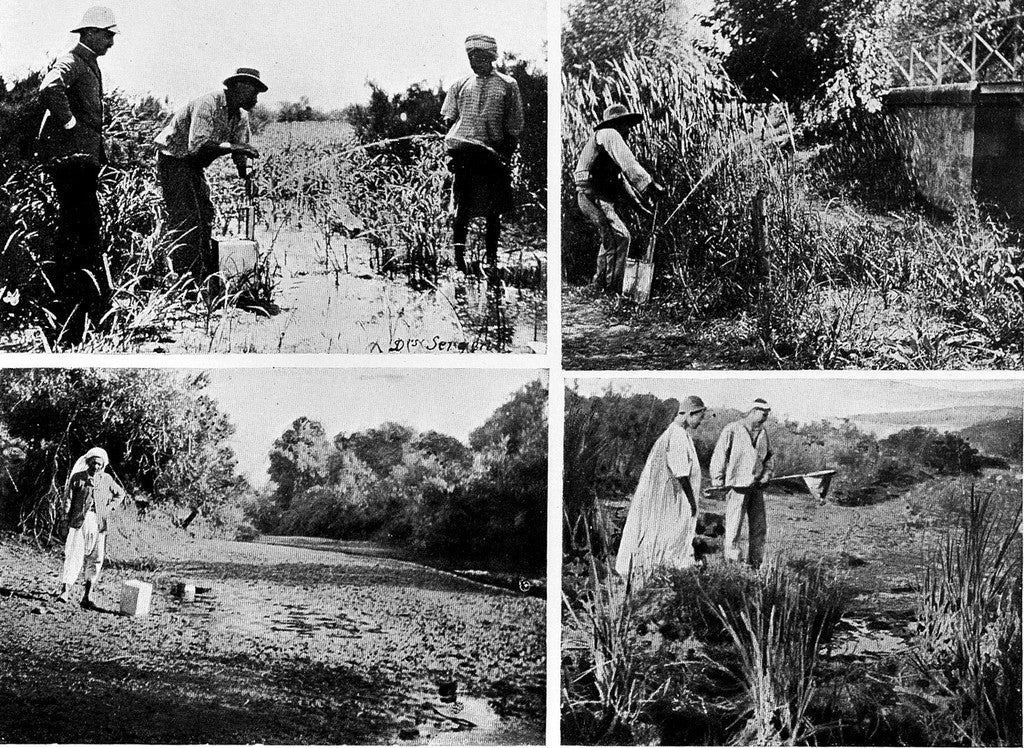
Laveran’s discovery pinpointed the parasite, but the mode of its transmission to humans remained unclear. For example, some scientists hypothesized that “the disease reached man in drinking water contaminated by parasites from the mosquitoes which rested on it. It took more work to elucidate that mosquitoes transmitted the infection by bite. That was explained and demonstrated by Sir Ronald Ross. An eldest of ten children, Ronald Ross was born in 1857, in India where his family has been living for some time. He trained for the Indian Medical Service, became interested in “sanitary matters,” and pursued bacteriology. Studying malaria in the native Indian population, Ross found that mosquitoes feeding on malaria victims contained the parasite in their tissues—and passed them onto those they bit. He later named the date he discovered this phenomenon, August 20, 1897, as the “Mosquito Day.” Both Laveran and Ross were awarded Nobel Prizes for their discoveries.
Forging Cures
Malaria may have devastated Italy, but ancient Chinese medics knew an effective remedy. For over 2000 years, they treated it with herbal tea made from Artemisia annua, a wormwood plant that contains artemisinin, which has antimalarial properties. This knowledge was lost, and later recovered from the ancient Chinese medicinal texts, turning artemisinin into a potent antimalarial drug. Similarly, South American tribes had their own remedy—a bark of the cichona tree. The European settlers learned the remedy from the South American natives, and introduced it to Europe around 1640, where it soon became a popular antimalarial remedy. Yet, it took chemists nearly 200 years to isolate the active anti-malarial compounds from the bark, which today we know as chloroquine—and which has been recently tried to treat COVID-19.
Because of medical breakthroughs and prevention efforts such as bed nets, insecticides, and repellants, the number of malaria-related deaths dropped by 40 percent between 2000 to 2014—from about 743 000 to 446 000. But then the progress slowed down. According to World Health Organization’s World Malaria Report 2019, the rate of infections didn’t decrease much from 2014 to 2018, and nearly as many people died from it in 2018 as the year before.
Weekly Newsletter
From polio to measles, vaccines proved to be an effective way to eradicate many diseases that plagued humankind. But making a vaccine for malaria was more challenging because the parasites have complex lifecycles and develop in different parts of the human body during different stages. At the early stage, parasites enter and mature inside the liver, and later replicate rapidly in the human red blood cells, causing fevers. So there are three types of vaccine scientists are working on. The first aims to prevent the parasites’ sporozoites or “eggs” from entering the liver, the second tries to decrease the number of parasites in the blood, and the third one attempts to stem the parasites’ development inside the mosquitoes themselves.
After 30 years of work, the first malaria vaccine was rolled out in Africa in 2019, despite concerns about it efficiency and safety. Named Mosquirix, it works by stimulating an immune response against a protein found on the sporozoites’ surface. Set up by WHO, the pilot effort aimed to vaccinate hundreds of thousands of children in Malawi, Ghana, and Kenya. The hopes are that it will make headway in humanity’s centuries-old malaria fight—but whether it does so remains to be seen.