For nearly a century, theoretical physicists have been grappling with a major foundational question: how does gravity work within quantum mechanics—the field devoted to the behavior and properties of particles smaller than atoms? The answer could open the door to a so-called “theory of everything,” one that explains the nature of both large objects and small particles, thereby bridging the gap between two fundamental physical theories, Einstein’s general relativity and quantum mechanics.
While there are many possible explanations—string theory arguably the best known—theoretical physicist Francesca Vidotto offers a theory that has the advantage of focusing on the specific question of quantum gravity: how gravity works on the quantum scale. Vidotto has spent much of her life unraveling and exploring this approach, which is called loop quantum gravity.
As Vidotto and fellow theoretical physicist Carlo Rovelli put it in their book, Covariant Loop Quantum Gravity, the “problem with quantum gravity is simply the fact that the current theories are not capable of describing the quantum behaviour of the gravitational field.” Many of us understand gravity—one of nature’s four fundamental forces—as the force that drives the dance of planets around other massive, celestial bodies. As children, we learn that it is what keeps our own bodies from flying off into space. Scientists once envisioned gravity as a traditional “force,” with the power to pull, but now, they understand it as a distortion of space-time.
But gravity does not translate easily into the mathematical language of quantum physics. It does not readily quantize. According to Vidotto, loop quantum gravity may be the key to understanding exactly how it works.
A successful theoretical framework for quantum gravity would help unravel many mysteries, from the beginning of the universe to the centers of black holes. Being able to merge general relativity—which works well on the cosmic scale—with quantum mechanics—which explains the workings of the miniscule scale—would provide answers that have hitherto been out of reach. We can’t yet observe what happens inside a black hole, but some theoretical physicists say quantum gravity could give us access to the “mouths” of these phenomena, where we know gravity is so great that it lets neither light nor matter escape.
Vidotto has been pondering questions like these since at least the age of 12, when she read Stephen Hawking’s A Brief History of Time. Riding her bike across the cobbled streets of her hometown in Treviso, Italy, she was struck one sunny morning with the conviction that questions about time, physics, and the universe were the kinds of questions she wanted to think about for the rest of her life. And so she has, partnering with giants in the field like Rovelli, her frequent collaborator, on theories like loop quantum gravity and spin foam.
Here, Vidotto shares thoughts on spin foam theory, her obsession with primordial quantum fluctuations, and one good way to turn kids into future scientists. She spoke to me from her new home in London, Ontario, where she teaches physics at The University of Western Ontario.
* * *
What drew you to science, in general?
When I was a little girl, I thought I would become a ballet dancer, or something else. During my first year of school this wonderful woman, my primary school teacher, had a method of teaching science to us that is still the way in which I think science works.
I remember her taking us out into the garden on the first day of school and asking us to draw pictures of what we saw in the garden. She asked us to look at our drawings and brainstorm about questions that were arising. We had to vote for the favorite question in the class. The question we chose was, “What are the rocks made of?” For the rest of the year we kept on studying this question together. We were divided into small groups. We had to make hypotheses. We had to collect this information set. We had to falsify our hypotheses. And this was wonderful.
For me, the most interesting part was that it was a very democratic process, where we listened to what the other groups of children were saying. The final answer to that question was in fact a collection of all the answers that were not falsified. And that’s where I saw a summary of the knowledge we had collected. So, every time I think about how to do science, what dynamics we put in place as scientists, the importance of listening to others and being free to possibly change our minds—these are lessons I learned when I was six years old and it’s something I bring with me to my work today.
Sounds like a good way to get more kids interested in STEM.
I think it’s really important. There is a lot of attention these days to get people interested in science, but I think we really have to get children involved in this process, this very interactive, engaging process of learning so they’re interested in science as they grow older.
Why are there so few women in science? The most effective actions are when girls are really young. There are studies that show how stereotypes get ingrained when girls are just eight years old, so we have to do something before that.
Which space and time question is always top of mind?
Well, I have an obsession now that has stayed with me since I started doing my own research: primordial quantum fluctuations. [You can think of primordial quantum fluctuations as the initial conditions before galaxies, planets, the universe came to be]. Today we have an understanding that all the structures we see in our universe, galaxies, stars and so on, all originated from some small seeds in the beginning of our universe. It’s a common understanding that this initial situation was of quantum origin. By running simulations where we include the presence of these fluctuations, we can recall where all the structures were that we now see.
We have a very good understanding of how big or which kind of fluctuation should be there, but we don’t have a theory that really provides them from scratch, from the bottom. So the theory I work with, quantum gravity, is a very promising candidate for a theory that provides a description of the quantum properties of space-time. In particular, it’s a theory that is able to describe these fluctuations, the quantum fluctuations that characterize space-time in the more extreme regimes, like in the beginning of the universe.
So my obsession since the beginning of my research was to try to use this theory, try to use quantum gravity, to study these fluctuations. This has been a long journey, but we have come to some important advances using numerical techniques. Of course, there are many projects and sometimes, I have to put this question aside, but I think this is the dream that will stick with me: to see really how we can describe the beginning of our universe using these techniques.
What is the primary discovery of loop quantum gravity theory?
Loop quantum gravity is a theory with the goal of describing the quantum properties of space-time. The main realization is the fact that, because space-time itself can be described as a geometry, geometrical quantities should appear with discrete values. So geometrical quantities are, for instance, area, volume, angle, and so on. If you try to measure these quantities at the fundamental level, you would see that they appear with only certain values and with jumps between those values. This is very similar to what happens in more familiar situations described by quantum mechanics. For example, when you study angular momentum in quantum mechanics, you discover that this property, if you try to measure it, comes only with certain values. And with jumps between them. This is the main discovery of loop quantum gravity, and everything stems from there.
Can you explain this idea of spin foam theory, an idea you’ve worked on with Carlo Rovelli?
Spin foam is simply the path integral formulations of loop quantum gravity. [Path integral formulation is the mathematical theory for understanding all the possible paths a quantum particle can take to get from one point to another.]
So loop quantum gravity is about describing the quantum geometry of space-time. The discovery of Einstein is that space-time itself, the geometry itself, is not something inert; it’s a dynamical object. It’s something that may evolve. Following this in loop quantum gravity, we want to describe how the gravitational field, the geometry, evolves, but in a quantum manner. The language in the quantum theory with which this is done is the path integral.
In quantum mechanics, for instance, you start with a particle. You see a particle at one point, and later you see the particle at another point. So you have an initial state with a particle in a given position and the final state with the particle in another position. Then you want to study what the probability is that the particle goes from one position to the other. In order to do that, you have to sum over all possible trajectories that the particle could have taken in order to go from that first position to the other. All these trajectories are the different possible paths the particle could have taken from the initial point to the final. Path integral is this idea that you have the sum of all possible paths.
Here in quantum gravity, what we do is study the probability to go from one state of the geometry to another state of the geometry. You have two states, but these two states represent a geometry, and you want to know what all the possible geometries are that interpolate from one to the other. In other words, all the possible configurations of the gravitational fields that take you from one configuration to the other.
This is spin foam. [Spin foam is a 2-dimensional model with labeled edges, vertices, and faces with the goal of describing the geometry of quantum spacetime. So named because it resembles a kind of foam.] It provides a concrete realization of how to compute this quantity, this sum of geometries. It tells you how geometry evolves, what the dynamics of the quantum gravitational field are.
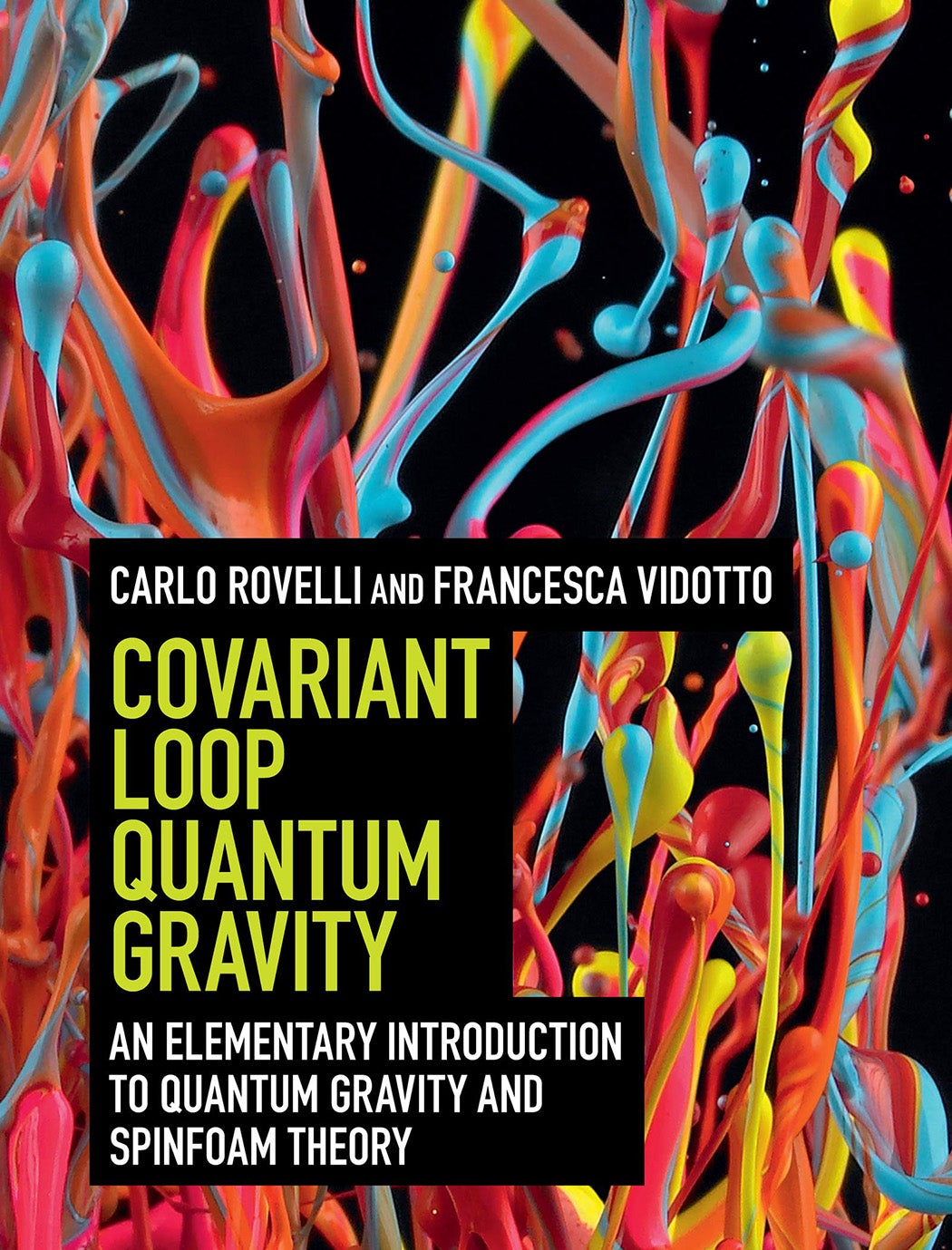
There are a select few who propose a merger between the two camps: loop quantum gravity and string theory. Do you believe there is compatibility between the two?
On both sides, we are theoretical physicists, we have been trained in quantum field theory and in general relativity, and we are using these basic tools in our work. Many specific techniques used by the two communities are similar, even if this is not always recognized. If you look at how different people conduct research on loop quantum gravity, you will find a lot of different techniques. We use certain terms and if you just check in the physics literature for string theory, you will find the exact same terminology. This means that both communities are somehow using the same things, and I think we are converging on the realization that some tools, some mathematical tools, are very useful to both sides. But we have different perspectives on how to implement them.
You can think of string theory and loop quantum gravity as one emerging from the particle physics community and the other emerging from the general relativity community. One camp stresses the importance of preserving the techniques that come from particle physics, while the other community stresses the importance of preserving what we have learned from general relativity. But, if we want to write down a theory of quantum gravity, we have to converge at a certain point.
String theory doesn’t really include a well-defined theory of quantum gravity. It was born to answer other questions, like unification of forces and naturalness and so on. As a by-product it was supposed to have a quantum theory of gravity in its belly. When evaluating string theory, you should be careful, because you have to be clear about what the questions are that you want to answer with that theory. With loop quantum gravity, it is easier, because loop quantum gravity is born to answer one question, that is, “How do we quantize gravity?” It’s a bit more of a humble question with respect to the other ones. I also think that because the question is more precise, we have been most successful with respect to that particular goal.
One fundamental property of the loop quantum gravity theory is that it allows for black holes to transition into white holes, which are the opposites of black holes, spitting out matter and energy, as opposed to consuming everything.
Oh, well, yes. The fundamental property is the fact that space-time is quantized, where you have fundamental grains of space-time. But this also has a counterpart, the fact that curvature is bounded. This means that the singularities that appear in general relativity are resolved in a quantum theory of gravity. Applied to the early universe, in cosmology there is a big bounce. The same thing happens in the center of a black hole.
Instead of having a singularity in the center, we just have a region that is very, very dense. When such a high density is reached, an effective quantum force appears like a repulsion that prevents the collapse of the black hole from continuing and triggers a new expanding phase that corresponds to a white hole. The idea that you can have a transition from a black hole to a white hole was something explored in the past, in the form of the Einstein-Rosen bridge [aka wormhole]. But the idea was that you would end up being in another region of space-time.
The insight that changes the picture is the possibility to have a transition to a white hole within our universe. Imagine you have some matter that starts collapsing, gets more and more dense, and in the process turns it into the very compact object that is a black hole. Then it reaches the maximal density and also a core of minimum size. At that point it starts to expand back, it’s as if you were seeing a movie in rewind, and everything starts expanding in the form of an explosion. If you can have things exploding back, this can also provide a possible window to search for quantum gravity, and we can look for traces of such an explosion.
So how does loop quantum gravity theory allow for the computation of the black hole lifetime?
The kind of computation that we are doing in quantum mechanics involves computing probabilities to go from one state to another. At this stage, we are configuring states of the geometry itself, so we have a state that corresponds to classical geometry. Take for example the classical geometry of a black hole. In other states, that corresponds to another classical geometry, which is the classical description of the white hole. The two states are, basically, exactly the same except for a flip of the curvature, one the inverse of the other with respect to the curvature.
In loop quantum gravity, we can write down the transition amplitudes, i.e. probabilities to go from one state to the other. While this is a quantum process, it can be connected to the time measured by an external observer for this process to happen. This research is something that is ongoing, so what is nice is that we have a theory to write down, concretely, these probabilities. Computing these discretions, these integrals, is complicated. One possibility is to try to solve these equations numerically, which is something we are doing now.
At this time, it’s not clear if we can have a transition to a white hole when the black hole is macroscopic—very big. But the probability becomes very high when the black hole is smaller, as in the case of a black hole that has undergone the Hawking evaporation and become very small. In that case, you really have a situation where the probability for this black hole to become a white hole is just one. It’s unavoidable. It’s possible you may end up having a very small compact object of Planck size that is in a superposition. They are black and white at the same time because they behave like quantum objects—so they can be in two states at the same time.
So this will be a manifestation of what it means for space-time to be quantized. In the quantum theory, superposition is one of the main features. For quantum gravity, being in a superposition means being in the superposition of different geometries. Here, I was giving you an example where you can really have a proposition of two different geometries, a black and a white geometry, together and in the same object.
How much influence do you think that different epistemologies have on our current understanding of theoretical physics?
Philosophy has always had a strong influence in the way in which we do physics. This was true for all of the great moments in physics. Both general relativity and quantum mechanics were born in a fertile philosophical environment, influenced for instance by the philosophy of Mach, or the philosophy of Poincaré. Einstein was even influenced by Schopenhauer. Those philosophers urged us to look at a world beyond the physics given by Newtonian mechanics. The twentieth century revolutions in physics were really inspired and fueled by philosophers.
Sometimes people don’t have a clear idea of what philosophy means. They have some romantic idea that philosophy is about the meaning of life, something like that. Instead, philosophy is a set of tools. The same way in which physics is a set of tools to think about the world using the language of mathematics, philosophy is also a sharp set of tools to distinguish clearly what the premises are of our way of thinking. It’s like a cleaning method for thinking.
I think that there is no physicist who does physics without being guided by some kind of philosophy. What I mean is that you should have a picture of what you’re doing, what your methods are, what your goal is, and what you want to understand. Without a clear philosophy, you risk doing bad physics. All the great physicists, of the past and of the present, had very good training in philosophy.
Can you share the philosophy that guides your own work within theoretical physics?
My way of professing in physics is not about the unification of forces, but I do have a drive towards looking for a common language, something that provides consistent features for all the different things that we have learned in fundamental physics. For me, what is more striking is how there is a form of relationality that emerges in all the different fields of contemporary physics. From quantum mechanics to general relativity to quantum field theory to quantum gravity, we understand better and better that all of the properties that we describe for physical systems always have to be related to something else. You can think about this “something else” as an “observer” or a “reference system.”
We always have to specify with respect to which system we are describing a given property, even things that are supposed to be at the foundation of our understanding of the world, like “things.” What do we call a thing? Do we call a thing a particle? Even the answer to questions about how many particles there are, how many particles a device detects, depends on the respective system we give this property. Then you start realizing that instead of thinking about the world in terms of things, it’s better to think about the world in terms of relations.
My philosophical background was also informed by the feminist philosophy of sciences. Feminist philosophers have been debating how you can have a notion of objectivity while there are so many different standpoints. From each standpoint, you have access to a bit of truth, but if you want to have a full description of reality, somehow again you have to accept this plurality of standpoints and eventually find a way to put them together. I believe this gives you a powerful key to interpret the world in which we live.
In the moment in which you confront yourself with what the “new” physics is telling you, there are two possible ways to react to this as a physicist. You can reject what nature is telling you, hanging on to some very rigid notion of truth and objectivity; or you may embrace it and try to look for the consequences of it. For me, embracing this second possibility is much more easy, and I see this as a very rich and fruitful way of thinking.
* * *
Glossary
Loop quantum gravity: A candidate theory of quantum gravity that focuses on loops or “discrete chunks” organizing the geometry of quantum spacetime.
Path integral: Path integral formulation, devised by American physicist Richard P. Feynman, is a mathematical theory for understanding all the possible paths a quantum particle can take to get from one point to another.
Primordial quantum fluctuations: The initial conditions that seeded the universe and everything in it, including the galaxies, planets, and stars.
Quantum gravity: A theory, still in progress, explaining how gravity works on the quantum scale. A universally accepted theory of quantum gravity could bridge the gap between quantum physics and general relativity.
Spin foam: A 2-dimensional model with labeled edges, vertices, and faces with the goal of describing the geometry of quantum spacetime.
White hole: White holes are theoretical opposites of black holes. They spit out matter and energy, as opposed to consuming everything.