The field of quantum mechanics dates to 1900, the year German scientist Max Planck (1858–1947) discovered that energy could come in discrete packages called quanta. It advanced in 1913, when Danish physicist Niels Bohr (1885–1962) used quantum principles to explain what had until then been inexplicable, the exact wavelengths of light emitted or absorbed by a gas of hydrogen atoms. And since the 1920s, when Werner Heisenberg (1901–1976) and Erwin Schrödinger (1887–1961) built new quantum theories, quantum mechanics has consistently proven its value as the fundamental theory of the nanoscale and as a source of technology, from computer chips and lasers to LED bulbs and solar panels.
One question, however, still puzzles: how does the quantum world relate to the more familiar human-scale one? For a century, the Copenhagen interpretation, chiefly developed by Bohr and Heisenberg in that city, has been the standard answer taught in physics courses. It posits that the quantum scale is indeterminate; that is, operates according to the laws of probability. This world is utterly different from the deterministic and predictable “classical” human scale, yet the Copenhagen interpretation doesn’t clearly explain how reality changes between the two worlds.
Heisenberg and Bohr developed the Copenhagen interpretation amidst the blossoming of new quantum theories in the first half of the twentieth century. In 1927, Heisenberg announced his important uncertainty principle: at the quantum level, certain pairs of quantities, such as momentum and position, cannot be simultaneously measured to any desired degree of precision. The more exactly you measure one, the less well you know the other. Thus, we can never fully know the quantum world, a key feature of the Copenhagen interpretation.
Indeterminism also appears in the Schrödinger wave equation at the heart of the Copenhagen view. Einstein had shown that light waves can act like swarms of particles, later called photons; in 1924, Louis de Broglie assumed the inverse, that tiny particles are also wave-like. In 1926, Schrödinger published his equation for these “matter waves.” Its solution, the “wave function” denoted by the Greek letter Ψ (psi), contains all possible information about a quantum entity such as an electron in an atom. But the information is indeterminate: Ψ is only a list of probable values for all the different physical properties, such as position or momentum, that the electron could have in its particular surroundings. The electron is said be in a superposition, simultaneously present in all its potential states of actual being.
This superposition exists until an observer measures the properties of the electron, which makes its wave function “collapse”; the cloud of possible outcomes yields just one result, a definite value emerging into the classical world. It is as if, asked to pick a card out of a deck, the instant you select the three of hearts, the other fifty-one cards fade away. In this case, we know that the rejected cards still physically exist with definite properties, but in the Copenhagen view, subatomic particles aren’t real until they’re observed. Another problem is that the notion of a sudden wave function collapse seems an arbitrary addition to the Copenhagen interpretation; it contradicts the smooth evolution in time built into the Schrödinger equation.
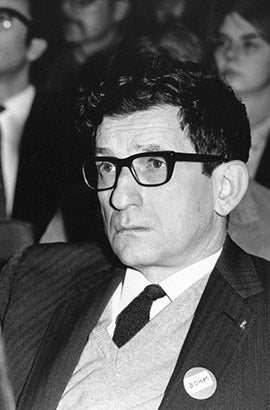
These troubling features, called “the measurement problem,” were hotly debated in the 1920s. But overwhelming any objections was the fact that the Copenhagen interpretation works! Its results agree precisely with experiments, the final test of any theory, and inspire real devices. Even so, David Joseph Bohm (1917–1992) and Hugh Everett III (1930–1982) sought equally valid theories without any incongruities. In the 1950s, these two American physicists dared to challenge the conventional Copenhagen interpretation with their “pilot wave” and “many-worlds” theories, respectively. Though from different backgrounds, Bohm and Everett shared characteristics that helped them seek answers: mathematical aptitude, necessary to manipulate quantum theory; and unconventional career paths, which separated them from the orthodoxy of academic physics.
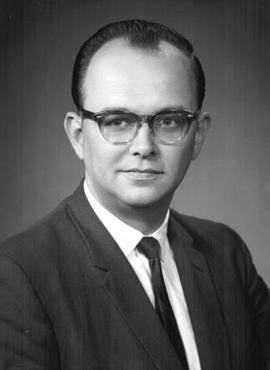
Bohm was a second-generation American, born into an immigrant family from Europe that operated a furniture store in Wilkes-Barre, Pennsylvania. In high school, where his physics instructor described him as “outstanding” and “brilliant,” Bohm developed his own alternative ideas about Bohr’s hydrogen atom. After undergraduate work at Penn State, he began earning a PhD in nuclear physics in 1941 under J. Robert Oppenheimer (1904–1967) at the University of California, Berkeley. The United States was engaged in World War II at the time and was about to build an atomic bomb. Bohm’s doctoral research was classified, and he was awarded his degree in 1943 without writing a dissertation. Though Oppenheimer wanted Bohm to work with him at Los Alamos, Bohm couldn’t get security clearance as he had briefly been, in the early 1940s, a member of the Communist Party.
In 1947, supported by theorist John Wheeler, Bohm became an assistant professor at Princeton. There he taught quantum mechanics and wrote Quantum Theory (1951), in which he presented the Copenhagen interpretation, only to disavow it the next year, when he published his alternative theory in a pair of papers in the Physical Review (in 1957, he expounded his ideas further in his book Causality and Chance in Modern Physics).
But in 1951, his life had taken a serious turn. In that Cold War era of McCarthyism, Bohm was brought before the House Committee on Un-American Activities (HUAC). He pleaded the Fifth Amendment against self-incrimination, and he was first indicted and jailed for contempt of Congress and then acquitted when the Supreme Court decriminalized this action. Still, the damage was done. Princeton didn’t renew Bohm’s contract and banned him from campus in June 1951. Unable to obtain a new academic position in the US, he began a life-long exile, taking temporary teaching positions in Brazil and elsewhere. Finally, in 1961, he accepted the offer of a chaired professorship in physics at Birkbeck College, London. He remained in that position until he retired in 1983, continuing to develop his new approach, the “pilot wave” theory.
When de Broglie postulated that tiny particles are also wave-like, he proposed the role of the waves as guiding or piloting the motions of real physical particles. Bohm fleshed out this insight by relating the pilot wave to Schrödinger’s wave function Ψ. In Bohm’s view, Ψ doesn’t collapse, but shepherds real subatomic particles into specific trajectories. This scenario yields the same results as the Schrödinger equation and resolves a great wave-particle quantum paradox. In the famous double-slit experiment, a stream of electrons or photons sent through two slits produces a pattern that could arise only from interfering waves, not particles. Bohm’s solution is that each particle traversing one of the slits rides a wave that pilots it into a complex path and generates an interference pattern from the swarm of particles.
For his part, Everett solved the measurement problem differently, as described by biographer Peter Byrne in an article, and later, a book. Born in Washington, DC, Everett showed an early interest in logical contradictions. At age twelve, he wrote to Einstein about the paradox “irresistible force meets immovable body,” and, as Everett reports, Einstein replied that there is no such paradox, but he noted Everett’s drive in attacking the problem. Everett graduated with honors from Catholic University as an engineer with strong backgrounds in math, operations research, and physics.
In 1953, Everett went to Princeton for graduate work. There he met Bohr, whose visit at the nearby Institute for Advanced Study sparked discussions about quantum mechanics. Later Everett said that the idea for his new theory came during a sherry-fueled session with one of Bohr’s assistants, among others. Everett was soon working out the consequences of his idea in a dissertation under John Wheeler, who had mentored Bohm and also Nobel Laureate Richard Feynman (1918–1988), and who called Everett “highly original.”
In the Copenhagen view, quantum reality as determined by the Schrödinger equation is separate from classical reality. Everett boldly asserted instead that the Schrödinger equation applies to everything, small or big, object or observer. The resulting universal wave function describes a reality without a boundary between microscopic and macroscopic or any need for the wave function to collapse. In his scheme, the measurement problem doesn’t exist.
This, however, comes at the cost of accepting a highly complex universe. If large objects and their observers obey the Schrödinger equation, then the universal wave function includes all observers and objects and their links in superposition. As Byrne explains: if the object could exist at either point A or B, in one branch of the universal wave function the observer sees the measurement result as “A,” and in another branch, a nearly identical person sees the result as “B.” (Everett called different elements of the superposition “branches.”) Further, without the jarring disruption of wave function collapse, the Schrödinger equation tells us that the branches go smoothly forward in time and do not interact, so each observer separately sees a normally unfolding macroscopic world.
In layman’s terms, this means that the universe, instead of being a unity that encompasses all reality, is filled with separate multiverses or bubbles of reality, each believed to be the entire universe by its inhabitants. The observer who saw result “A” now lives in that reality, and the person who saw “B” occupies a separately evolving reality according to their different outcome. Each of these unimaginable numbers of bubbles moves ahead into its own future, forming a totality filled with what have come to be called “parallel worlds.”
Bohr and his group scorned this grandiose idea as an answer to the measurement problem, one of his circle calling it “theology,” and another deriding Everett as “stupid.” Wheeler had Everett rewrite his dissertation so it didn’t directly criticize the Copenhagen interpretation or its proponents. His thesis was published in 1957 and, according to Byrne, “slipped into instant obscurity.” All this should have been no surprise. As Olival Freire Jr. points out, Bohm’s earlier work—which Everett cited—had also been badly received, even with hostility, in a community dominated by Bohr and champions of the Copenhagen interpretation.
That was to change for both theories. In 1964, a bombshell result from theorist John Bell showed how to experimentally confirm the exceedingly strange quantum effect of entanglement, which Einstein called “spooky action at a distance”: the fact that two quantum entities can affect each other over arbitrary distances. Bell, it turns out, was strongly influenced by Bohm’s work, notes Freire. This shows that rethinking the foundations of quantum mechanics, downplayed by some physicists as only a philosophical exercise, can pay off in deep theoretical insights as well as in technology; entanglement today is used in quantum computation, communication, and cryptography.
Everett’s ideas too came to be more appreciated after The Many-Worlds Interpretation of Quantum Mechanics (Bryce DeWitt and Neill Graham, editors) was published in 1973. It included Everett’s original dissertation and related papers. This and DeWitt’s evocative phrase “many-worlds interpretation” brought new interest in Everett’s work and linked it to multiverse theory, which has been developed to solve certain problems in cosmology and as an outcome of string theory. Everett won further recognition—this time in popular culture—in 1976, when his work appeared in Analog, a leading science fiction magazine. (In fact, multiverses and parallel worlds have become staples of popular culture, as the film Everything Everywhere All at Once (2022) and the streaming series Dark Matter (2024), based on the novel by Blake Crouch, make clear.)
By 2023, Bohm’s and Everett’s seminal papers had each amassed tens of thousands of citations in the scientific literature. Surveys have also asked hundreds of physicists which interpretation of quantum mechanics they consider best. Many chose the Copenhagen view, but an equal number favor either the pilot wave or many-worlds interpretation. It’s striking that what in the 1950s were outlaw ideas, met with disbelief and antagonism, today have a significant degree of acceptance and have greatly expanded our view of the quantum world and the universe.
That Bohm and Everett could produce novel theories reflects their special circumstances and their times as well as their abilities. McCarthyism interrupted Bohm’s career but also freed him from conventional views of quantum mechanics. Historian of science Christian Forstner cites a 1981 interview in which Bohm acknowledges the upside to his departure from Princeton. It “liberated me,” Bohm admitted. “I was able to think more easily and more freely…without having to talk the language of other people.” Forstner notes that in exile, the physicist had the freedom to choose like-minded colleagues so that “the US-community and its thought-style lost importance for Bohm.” Indeed, Bohm’s exile was highly productive.
Weekly Newsletter
Everett’s expertise in operations research brought him the offer of a position with the Pentagon’s Weapons Systems Evaluation Group (WSEG) to analyze nuclear warfare after finishing his PhD. Wheeler wanted him to continue at Princeton but also knew, Byrne writes, that the lack of recognition for Everett’s ideas had left him “disappointed, perhaps bitter.” Nor did Everett enjoy truncating his thesis to mollify Bohr, and he must have realized that advocating an unpopular theory would cloud his academic career. In the end, he chose WSEG and never again worked in theoretical physics, but perhaps having this alternate possibility stiffened his resolve in presenting and defending his audacious idea. His talents shone at WSEG, but, according to Byrne, he was an alcoholic and died of a heart attack at age fifty-one.
The co-existing Copenhagen, Bohm, and Everett interpretations give the same results for many different tests of quantum behavior; and so we await the subtle experiment that distinguishes among them, showing which one is physically true and might give philosophers new insight into the nature of reality. Bohm’s and Everett’s sagas provide another valuable lesson. Science prides itself on being self-correcting; wrong theories are eventually made right, as in the old notion of a geocentric universe giving way to the modern view. The Copenhagen interpretation became unquestioned orthodoxy, but Bohm and Everett challenged it even at personal cost. That reflects the highest aspirations of science and deserves to be recognized in 2025, the upcoming International Year of Quantum Science and Technology.
Support JSTOR Daily! Join our membership program on Patreon today.